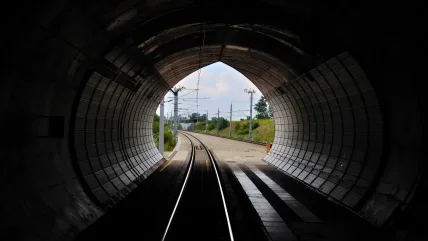
Open face techniques such as the sequential excavation method (SEM) combined with sprayed concrete lining (SCL) allow rapid tunnel or shaft advancement in stiff cohesive clay soils. In these conditions the exposed face typically remains stable for a few hours while the sprayed concrete is applied and reaches design strength before the next advance. Where a heading interacts with an aquifer resulting in water bearing granular soils in the face there will be an obvious risk of groundwater inflow leading to ground loss and face instability.
The benefits of open face tunnelling in terms of speed and flexibility are such that there can be significant advantage in controlling groundwater levels sufficiently to allow their application in more marginal conditions. Interfaces and boundary conditions are two characteristics of the interaction between aquifers and excavations which are frequently misunderstood and which between them often dictate the strategy and control the viability of dewatering for tunnels and shafts.
Marginal conditions for open face tunnelling often comprise predominantly cohesive soils with one or more water bearing granular horizons which may have an upper confining layer or a lower low permeability horizon or both.
Figure 1 illustrates a range of hydrogeological settings with a planned tunnel in close proximity to a sand aquifer which is either unconfined, confined or a thin confined aquifer. In each case the interface between the low permeability horizon and the aquifer can be above the crown, within the face or below the invert. These scenarios illustrate several issues as follows.
- For Settings A, F, G, and I, the water-bearing horizon does not intersect the tunnel. The tunnel temporary works designer will need to check whether there is a requirement to control pore pressures in the water bearing granular layers during the tunnel works. If there is a need, it would be necessary to specify the target pore pressures for any horizon in the vicinity of the tunnel.
- For the remaining Settings, B, C, D, E, and H, the aquifer intersects the tunnel, and full reduction in pore pressure to the base of the tunnel or base of the water-bearing horizon will be required to maintain stability. For three of the settings, B, C, and D, the crown of the tunnel is in non-cohesive soil, and even if dewatered additional support measures, such as roof spiles, may be needed to support the crown during each advance.
- It is generally relatively straightforward to control groundwater levels by dewatering where there is a reasonable thickness (>1m or 2m) of the aquifer below the tunnel invert, as in Scenarios C, D, E, F, and I. This is because wells can be installed downwards from the surface or tunnel to target the aquifer with a target drawdown either to below invert, Scenarios C, D, and E, or perhaps above invert for Scenarios F and I where the cohesive soils present below invert are able to act as a plug and resist some uplift pressure.
- It is less straightforward to control groundwater levels where there is a sand/clay horizon, referred to as interface conditions, within the face or in very close proximity, Scenarios B and H. This is because the target drawdown level is the base of the aquifer, which generally requires close well spacing to reduce inevitable ‘overbleed’ of groundwater at the interface to tolerable conditions.
- For Scenarios A and G, the water-bearing horizon is above the crown.
Whilst it may be feasible to control pore pressures it is important to appreciate that generally some residual groundwater will remain above the sand/clay interface.
Figure 2 shows the exposed face of a cross passage excavation with London Clay above axis level and Harwich Formation/Lambeth Group Sands below axis, which extend to approximately 3m below invert level. This is a clay/sand interface, Figure 1 Scenario E, which is relatively straightforward to manage, in this case with an array of 15 surface deepwells readily achieving the 23m of drawdown required.
Geotechnical engineers generally grasp the need to assess the permeability of a target aquifer horizon and its significance in the design development of a groundwater control strategy. Some indications of the permeability of a granular horizon can be derived from sample descriptions, particle size distribution testing, natural gamma radiation measurements or in-situ variable head tests. Often less well understood is the significance of the aquifer boundary conditions, which can usefully be considered as the recharge or sources of inflow (and sometimes outflow) to an aquifer. For an unconfined aquifer, this is likely to be a combination of precipitation and leakage flow from streams, rivers, estuaries, ponds/lakes, or adjacent aquifers.
Recharge to a confined aquifer is likely to be more remote, perhaps from where the aquifer becomes unconfined. Confined aquifers – particularly thin, confined aquifers – can sometimes have very remote or limited sources of recharge which can significantly simplify the required extent and capacity of a groundwater control scheme. This is illustrated in Figure 3 which shows a sand horizon interacting with a tunnel heading. The sand horizon is the same level and thickness for each case but with different boundary conditions.
Figure 3A shows an aquifer of limited lateral extent so has barrier boundaries with minimal recharge; once drawn down inflows will be minimal and recovery of pore pressures when pumping is stopped will be slow. Figure 3B shows an extensive sand horizon with a distant source of recharge which will have persistent inflow and faster recovery than 3A.
In Figure 3C the sand horizon links to a thick gravel horizon providing a close and potentially significant source of recharge. In this case inflows will be greater and it may be challenging to achieve acceptably stable conditions at the interface.
It is important to appreciate that boundary conditions cannot be assessed from a geotechnical investigation comprising logged boreholes, soils samples, laboratory testing, piezometer monitoring, and in situ variable head tests or packer tests.
Aquifer boundary conditions can only be assessed from a hydrogeological investigation, which includes an appropriately designed pumping test comprising one or more test wells and an array of piezometers.
The piezometer response to pumping on the wells is controlled both by the aquifer parameters, primarily the permeability, but also, providing the pumping stresses the aquifer sufficiently, by the boundary conditions.
Had the cross passage in Figure 2 been a few metres lower in the geological sequence then we might have been faced with the potentially more challenging Figure 1 Scenario H with a clay/sand interface in the face. A possible strategy for this situation would be to scale back the surface well scheme and introduce in-tunnel wellpoints targeting the sand/clay interface each side of the cross passage. Note that the presence of the TBM drives at each end of the cross passage may act as a cut-off boundary for the aquifer between, curbing inflows and simplifying the dewatering arrangements.
In summary interfaces and boundary conditions are relatively simple concepts which can help develop the conceptual model, characterise the interaction between a tunnel or shaft and aquifer, and provide focus for a dewatering strategy and associated geotechnical and hydrogeological investigation.